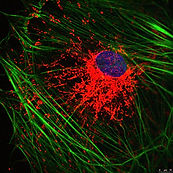

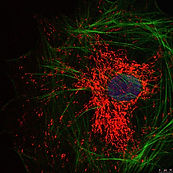
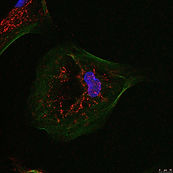

Mitochondrial Multitudes: Quantifying Mitochondria in Human and Cuvier's Beaked Whale Fibroblasts
By: Natalie Kubicki (Trinity '23)
January 2022
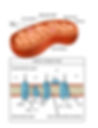
Mitochondria Overview
In introductory biology courses, mitochondria are rarely covered beyond their “powerhouse of the cell” taglines. However, what are depicted as bean-shaped organelles in every textbook, are actually complex, interconnected tubular mitochondrial networks coordinating cellular energy.
One of the main functions of mitochondria is producing ATP, the energy of cells, through oxidative phosphorylation. Oxidative phosphorylation is the passing of electrons down the electron transport chain, which produces an electrochemical gradient across the inner mitochondrial membrane. The energy stored in the gradient is then used to make ATP in a process called chemiosmosis. But why is all of this relevant to marine mammals and hypoxia? Oxygen plays a huge role in oxidative phosphorylation! Oxygen accepts the electrons at the end of the electron transport chain and is key in keeping up the production of ATP. Also, when electrons are not correctly passed through the electron transport chain, oxygen can be partially reduced and form Reactive Oxygen Species (ROSs). Low levels of ROS are important for adaptations seen in hypoxia, and high levels of ROS activate cell death pathways. Therefore, in hypoxic conditions, the mitochondria must adjust to regulate ATP levels and manage ROS production. Deep-diving marine mammals’ abilities to be highly functional with very little oxygen elucidates that there may be significant differences between human and marine mammal mitochondrial adaptations.
Image Analysis
There are many different levels on which cellular adaptations can be examined in response to hypoxia, but we started with cell composition. Driven by the question “how does the quantity of mitochondria compare between normal human dermal fibroblasts (NHDFs) and Cuvier’s beaked whale fibroblasts?”, we compared mitochondrial quantity through fluorescent microscope images.
In these images, mitochondria are stained with TOM20-Cy5, making the mitochondria fluoresce red. The nuclei are blue and the F-actin is green.
It was observed both qualitatively (by direct observation) and quantitatively (ImageJ) that there are significantly more mitochondria in the whale fibroblasts than in the NHDFs.
In order to quantitatively analyze the mitochondria, ImageJ was used. See the explainer video below to see the ImageJ process!
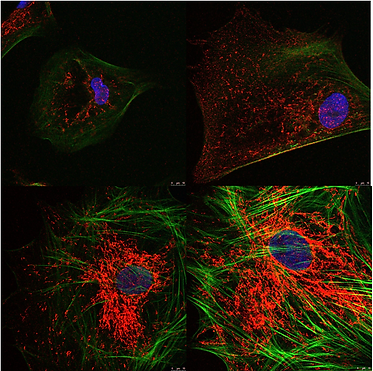
First Row: 2 side-by-side human fibroblasts
Second Row: 2 side-by-side Cuvier's beaked whale fibroblasts
ImageJ Explainer
What's Next?
So, what’s our next question?
​
There are many future directions for the comparison of mitochondrial function between human and deep-diving marine mammals. Experiments can be performed on the levels of genomics (DNA), transcriptomics (RNA), proteomics (proteins), and metabolomics (metabolites).
​
For example, on the genomic level, we can investigate if genes in the nuclear genome that are related to mitochondrial function are overexpressed in deep diving marine mammals. If we “knock-out” (make not functional) these genes in marine mammal cells, we could examine if there is a different hypoxic response. On the metabolomic level, we can compare the products of processes such as oxidative phosphorylation and glycolysis between humans and whales. If they are different, we could relate these deviations back to mRNA/protein level activity.
These are just a few of the endless possibilities of investigating mitochondrial relation to hypoxic pathways. Mitochondria really are the powerhouses of the cell!
Further Reading
​
Formation and Function of Mitochondrial Networks:
​
Hoitzing, H., Johnston, I. G., & Jones, N. S. (2015). What is the function of mitochondrial networks? A theoretical assessment of hypotheses and proposal for future research. BioEssays : news and reviews in molecular, cellular and developmental biology, 37(6), 687–700. https://doi.org/10.1002/bies.201400188
​
Genetic Alterations of Metabolism in Hypoxia-Tolerant Mammals:
​
Tian, R., Yin, D., Liu, Y., Seim, I., Xu, S., & Yang, G. (2017). Adaptive Evolution of Energy Metabolism-Related Genes in Hypoxia-Tolerant Mammals. Frontiers in genetics, 8, 205. https://doi.org/10.3389/fgene.2017.00205